Success Stories
With the help of bioengineering, we can process waste and turn it into substances of higher value, such as bioplastics, says Pavel Dvořák
Our goal is to modify the metabolism of microorganisms so that they can produce useful substances, such as bioplastics, biofuels or biopharmaceuticals.
doc. M.Sc. Pavel Dvořák, PhD
associate professor
Microbial Bioengineering Laboratory, Microbiology Department, Department of Experimental Biology
Pavel Dvořák is currently an associate professor and head of a research group at Masaryk University (MU), where he also received his M.Sc. (2009) and Ph.D. (2014) in Molecular and Cell Biology. He began his research career at the Loschmidt laboratories by engineering a synthetic enzymatic cascade for the degradation of a halogenated anthropogenic pollutant. He completed his postdoctoral internship (2015−2018) in the laboratory of one of the European pioneers of synthetic biology, prof. Víctor de Lorenzo, in Madrid. While there he received a two-year Marie S. Curie scholarship for a project focused on engineering the metabolism and cell surface of the bacterium Pseudomonas putida for the biotechnological processing of carbohydrates from waste plant biomass. In 2019, he returned to MU and founded the Microbial Bioengineering Laboratory.
Photo: Irina Matusevich
What profession did you dream of as a child?
It wasn’t science😊! As a little boy, though, I told my parents that I wanted to be a detective, which is actually not that far from science - you are also looking for evidence to confirm a hypothesis. Also, I believe that a good scientist, especially if they are leading a team, must also have a strong sense of justice.
How did you end up in the natural sciences?
My interest in the natural sciences, especially biology and chemistry, developed from elementary school through high school in my home town Kroměříž. In both places, I was lucky to have very good biology teachers who supported me in various extracurricular activities, such as biological and ecological Olympiads, Secondary School Vocational Activities (Středoškolská odborná činnost) etc. I was also drawn to nature from an early age. When I need to calm down or relax, I run to the forest, whether with family, friends or alone − and I mean “running” literally, because running is my other hobby besides nature. But today it is only recreational.
You focus on the field of synthetic biology and methods of genetic engineering. Please explain to the readers what you aim for in this field?
Our goal in general is to introduce engineering practices into biology to modify the regulatory and metabolic pathways of living microorganisms. We do this by making changes in bacterial producers using genetic engineering techniques at the DNA level. It’s not magic, these techniques have been around now for fifty years, they just keep getting better. The techniques performed in our laboratory are similar to the evolutionary processes that have been taking place in nature for billions of years. Just as humans have been breeding more resistant and more profitable types of wheat or cattle for thousands of years, today we “domesticate” and “breed” bacteria and yeast in the laboratory for more efficient production of medicines, biofuels or even new materials.
A textbook example is the biotechnological production of insulin, which has been produced by genetically modified bacteria and yeast since the 1980s. Could you explain to the readers the synthetic biology techniques used?
Scientists isolate the gene encoding the protein insulin from human DNA in the laboratory and insert this gene into the bacterial DNA molecule in a test tube using molecular “scissors” and “glues”, i.e. catalytic protein enzyme molecules that can “cut” and “glue” DNA (by the way, these are also isolated from bacteria). The bacterium accepts this gene and learns to "read" it and transcribe it into the structure of the insulin protein. This is possible because the letters of the DNA alphabet, the alphabet of life, are universal, they are the same in all organisms, and therefore one organism can read a gene from another organism. In nature, it is quite common for microorganisms to “exchange” different genes with each other and use them as they adapt to new conditions.
In 2019, after your return from a long-term postdoctoral internship abroad, you founded the Microbial Bioengineering Laboratory, which you lead. The laboratory operates within the Section of Microbiology of the Department of Experimental Biology at the MU Faculty of Science. What organisms are you specifically targeting?
In our laboratory, we study microorganisms - specifically bacteria - and their rich metabolism, as well as the possibilities of using these bacteria in modern biotechnologies. We try to emphasise that microorganisms are not just the causative agents of infectious diseases but that they have been helping humans in many ways for thousands of years, and that thanks to modern technologies, they can help even more. We try to adjust their metabolisms. Our goal is for them to be able to produce useful biomaterials, biofuels or biopharmaceuticals. That is the ideal end-point; on the way, we undertake basic research on the microorganisms.
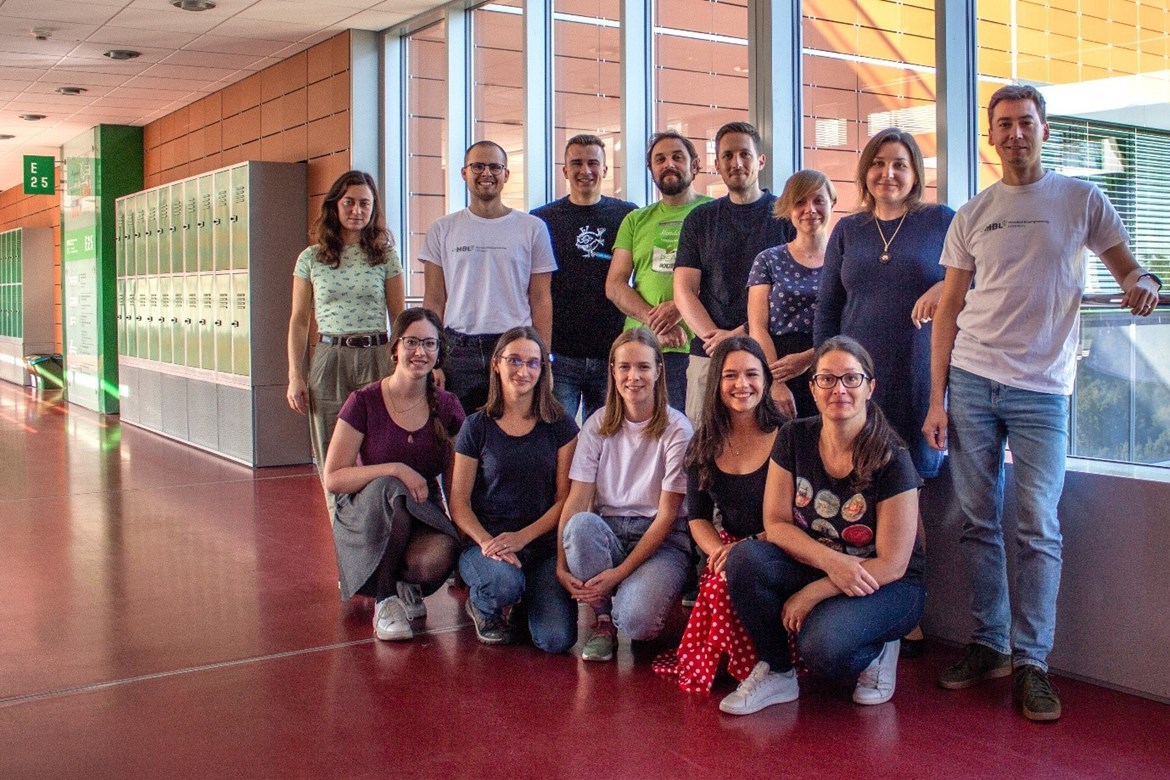
How does the metabolism of a single-celled organism actually work? Can you describe it?
If you imagine that the biochemical pathways in a cell are pipelines leading from the input substance (or substrate as we call it), which may be sugar from plant biomass, then the metabolism pipeline breaks down these input substances into basic building blocks. From these, the cell builds its body and can produce interesting chemicals - but it doesn’t do this in large quantities. We are trying to modify this “pipeline” so that the cell produces interesting chemicals in quantities that will be of interest from an economic point of view. We focus especially on the metabolism of sugars, i.e. that part of the “pipeline” that breaks down the substrate sugar (e.g. glucose, xylose) into other building blocks.
Where does the food (in your case sugar) for the cells to perform this biological synthesis come from?
Until now, the sugar was mainly obtained from starches, the material that you get after grinding maize, wheat or potatoes; however, this is problematic as it is wasteful. Today, therefore, we try to use waste materials as input substrates in biotechnological operations. We focus on waste plant biomass and its indigestible component, lignocellulose. The agricultural industry only tends to process the cob from maize, and we are interested in everything that is left over, i.e. the stalks and leaves. Much of this is used as fertiliser or feed, but around a third remains that can be used as a cheap input for biotechnology. Our goal is to process waste and turn it into something valuable, a process known as “trash to treasure”.
What genetically modified bacteria do you work with?
My team works with the bacterium Pseudomanas putida, which has been studied in relation to biodegradation and bioremediation of toxic waste since the 1970s. Interestingly, it was the first patented genetically-modified organism in the world. The path to obtaining a patent was not at all easy as it was something completely new. Dr. Ananda Mohan 'Al' Chakrabarty, who helped modify the bacterium in the USA, was able to insert the genes of other bacteria into the pseudomonad and improve its ability to degrade petroleum products. Since then it has been used in biotechnology.
What makes this pseudomonad so interesting to you as a synthetic biologist?
It is very resistant to the various stresses encountered in nature, and is often isolated from sites with oil or toxic environmental contamination. It can do this thanks to aspects of its metabolism that help it transform these ‘toxic’ chemicals into substances that are less toxic or that it can use for growth. Its metabolism “pipeline” is very rich and diverse and it easily adapts to demanding conditions. We now know that it can be used not only for the removal of toxic substances from the environment but also in bioproduction, especially where the conditions for organisms in large fermenters are very stressful. To put it simply, these organisms did not evolve to swim in ethanol, but we modify them to be able to do it. It is this robustness and resistance that is so attractive for synthetic biologists.
This summer, you and your team received the prestigious “Jay Bailey Young Investigator Award” for promising young researchers from the International Metabolic Engineering Society (IMES) for both your pseudomonad research and other research projects mentioned below. Congratulations😊. What made your research so interesting?
I started working with this pseudomonad during my postdoctoral internship, using it to process various sugars in lignocellulose from waste plant biomass. I was the first to prove, and publish, that it can process multiple sugars from the biomass at the same time. It is also exceptional among similar organisms in that it can process lignin, a component of lignocellulose, which is made up of aromatic and often toxic substances which this bacterium seems to enjoy. Colleagues from the USA, who followed up on our study, showed that it can also process the sugars and toxic substances at the same time. Most organisms cannot do this, but after our modification, this pseudomonad was able to do it. Theoretically, therefore, this considerably shortens the time needed to process sugar from waste lignocellulose, and thus also reduces the financial costs. Our colleagues abroad, including those at the National Renewable Energy Laboratory in Colorado, followed up on our work and took it further. This resulted in a pseudomonad variant capable of converting sugars and aromatics from waste lignocellulose into useful molecules such as muconic acid, a chemical precursor to nylon. Our colleagues work is already so far advanced that they could move to the commercial phase within a few years. I am glad that we contributed to this advance. This was one of the reasons why we received the award, for which I was nominated by Gregg Beckham from the National Renewable Energy Laboratory in Colorado, among others.
Are you still studying this pseudomonad?
Yes, we are still working on its metabolism and investigating it in more depth. We now have a study in the publication process where we use omics technologies to look at the evolution of mutant bacteria that we have prepared for better growth on xylose, an important sugar from waste plant biomass. We are watching how this bacterium develops better growth thanks to our targeted metabolism interventions, natural evolution, which we encourage through the modifications, and better processing of a substrate that it does not normally consume but which we "taught" it to eat. However, large multidisciplinary teams, sometimes entire institutes, are working constantly on biotechnologies in order to reach the commercial stage; we individually do not have either the resources or the ambition to do this at the moment. Nevertheless, we contribute to this process by learning in detail how the bacteria’s metabolism works and by developing methods to improve it through targeted interventions.
But you are also currently researching thermophilic bacteria. What potential do they have in the biotechnology field?
Extremophilic, or more specifically thermophilic, bacteria can handle high temperatures. Pseudomonads grow at lower temperatures around 30 °C. With today’s modern biotechnologies, however, many of the chemical processes could work more efficiently at higher temperatures. We work with those bacteria that have an optimal temperature range of 50 to 60 °C. These also have great potential because there is a lower risk of production contamination by mesophilic species at higher process temperatures.
You mentioned that your research is always interdisciplinary. Who are you collaborating with on this new research? What kind of experts do you need?
We are presently working with prof. Stanislav Obruča from the Faculty of Chemistry at Brno University of Technology (FCH BUT), Dr. Karel Sedlář from the Faculty of Electrical Engineering and Communication Technologies BUT (FEKT BUT) and doc. David Šafránek from the MU Faculty of Informatics (MU FI). We have created a unique consortium of laboratories that complement each other with knowledge, methods and equipment. We specialise in both synthetic and molecular biology. Mathematical models, which are provided by colleagues from FI MU and FEKT BUT, play a huge role in our research, and the BUT chemists have fermenters and experience in processing cells, input substrates and final products. We are trying to prepare genetically modified bacteria that will be able to produce polyhydroxyalkanoate (PHA) biopolymers rapidly and in large quantities from cheap waste substrates. To do this, we modify the bacteria’s genetic information so that it can consume various sugars from cheap waste lignocellulose and direct the carbon from these cheap sugars into the formation of PHA as efficiently as possible through its metabolic pathway pipeline. In our latest study, we show that all that is needed for a more efficient flow of carbon from sugar through the first part of the metabolic pipeline is to open a new efficient pathway for sugar to enter the cell.
This new strategy of metabolic engineering, which can also be used in the production of bioplastics, is a great success story for your laboratory. First, tell us how and why bacteria, whether natural or modified, produce bioplastic?
Several natural bacteria like sugars, and these can store excess carbon from the sugar in intracellular polyester granules, called polyhydroxyalkanoate (PHA). These PHA molecules are very diverse, depending on which sugars or other substances the bacteria consume. Importantly, they have properties similar to synthetic polymers, i.e. synthetic plastics made from petroleum. However, unlike synthetic plastics, PHAs are completely degradable in nature, are non-toxic, come from renewable sources and are biocompatible. This means that they can be used to make artificial implants used in medicine, for example, or threads for suturing wounds, and so on. They can also serve as packaging materials or as cloche tunnels used in agriculture. There are really many possible applications. Unfortunately, the price of PHA is still too high compared to the price of synthetic plastics, therefore their production is only a fraction of a percent of all plastics that are produced globally.
How do you do these metabolic engineering modifications?
We insert a gene encoding a sugar transporter protein into the selected bacterium, which then transfers a sugar molecule from the external environment into the cell. That transporter has a very high sugar transfer capacity, and that sugar, to put it simply, is pushed into the bacterial cell, which then reacts to it by overproducing molecules. After further modifications, bioplastics can be formed more efficiently from these molecules. We recently published this research in the prestigious international journal Metabolic Engineering.
Your student, Barbora Hrnčířová, is very successful. With its research on the decomposition of waste plant biomass, it also attracted experts from NATO, as we wrote here... So does your laboratory also involve students in research?
Yes, our students are also involved in various parts of this large project. Barbora Hrnčířová is trying to modify selected bacteria so that, in addition to simple sugars, they can also consume more complex sugars. The sugars in lignocellulose are stored as polymers, you can think of these as beads on a string, with the simple sugars as individual beads. Most bacteria can only consume individual small beads - simple sugars. To get them, you must first break the chain of beads. To do this, biotechnologies use either chemicals, such as acids or bases, high temperatures or catalytic proteins and enzymes. The process is very expensive and energy intensive, which makes the production of PHA more expensive. Bára and some other members of our team are trying to get our small bacterial PHA producers to consume even longer chains of sugars, or to cleave those chains themselves using their own catalytic proteins, which we introduce into them using bioengineering techniques. This should lead to a more efficient and cheaper process of converting waste plant biomass into desirable chemicals such as PHAs and many others. She recently presented this concept at the NATO Science and Technology Organisation meeting in Helsinki and won the award for best presentation. At the same time, she was the only presenter with a biological topic.
Finally, tell us what challenges you face in your teaching activity?
The main challenge for me is the possibility of engaging a wider spectrum of listeners for what I am currently lecturing on. Not all students who come to a lecture are really interested in the given subject or the topic of a specific lecture (this applies mainly to compulsory subjects that students must complete). I try to present the material in such a way that everyone in the auditorium can understand it. This also makes it more attractive. To me, the amount of work or the number of slides or methods that the students remember is not so important, instead, I want them to remember the principle, the central idea. Practical demonstrations and personal experience from the laboratory are also important. No amount of theory can replace that. I believe that, overall, we should put more emphasis on practical teaching, seminars and solving practical problems. I also consider a personal approach to students to be very important. However, again, this is possible with a limited number of listeners in a class or students in a research group. So, we’re back to quality over quantity.
Thank you for the interview
Zuzana Jayasundera
Translation: Kevin Frances Roche